Gravitational waves were predicted as far back as 1916 by Albert Einstein in his theory of relativity. His calculations showed that massive interstellar objects such as black holes or neutron stars that orbited each other had the ability to cause ripples that would disrupt space-time. These waves are usually caused by black holes colliding or when large stars (supernovas) explode towards the end of their life. This ripple, though invisible, moves at the speed of light and stretches and squeezes any object/s in their path. It has also been predicted that the great Big Bang has also created these waves. In a sense, gravitational waves create disturbances and ripples in the space-time continuum.
He first speculated that anywhere near a celestial body such as the Sun or a planet, there would be a curve or disturbance in the space-time environment. This was true for static bodies. He pushed his theory further and sought to find out what would happen if the body were to move and/or shake. He found that ripples would occur similarly to how it happens when children jump on a trampoline. Though Einstein predicted their existence, he continually published papers that refuted this theory and went back and forth on their existence.
In the 1960s, scientists tried to find evidence of the existence of gravitational waves. Joseph Weber from the University of Maryland was one of the first to try. He used giant solid aluminum cylinders, hoping that they would be able to detect gravitational waves that would pass through them. After 10 years he recorded success. He claimed that these detectors would ring almost daily. This led to collaboration with other scientists, but even after this, not even a single wave was detected.
The first proof of these waves came in the year 1974, fifty eight years after Einstein predicted their existence. In the observatory of Puerto Rico using the Arecibo Radio Telescope, two astronomers observed a binary pulsar. This was the type of object that would have been seen from the radiation after gravitational waves. To definitively confirm that this was a product of gravitational waves, they observed the two stars orbiting each other in the area where the pulsar was seen. This observation took 8 years, and they found that these stars were moving towards each other at the rate predicted in the theory of relativity if stars were to emit gravitational waves. This discovery earned Joseph Taylor and Russell Hulse the 1993 Nobel Prize.
After this discovery and conclusion, several other astronomers were able to observe and study pulsar radio emissions, coming to the same conclusion. This proved the existence of gravitational waves, although only mathematically.
In 2015, the greatest discovery was made. Scientists were able to directly detect gravitational waves for the first time. Using the Laser Interferometer Gravitational-Wave Observatory (LIGO), they detected an oscillation in space-time that had been caused by gravitational waves. These waves were caused by two colliding black holes that were 1.3 billion light years away. This was one of the greatest scientific discoveries ever made. It went further in clarifying the fact that Einstein was right. These gravitational waves were created by two orbiting black holes. These black holes were 29 and 36 times the mass of the Sun. As they orbited around each other and were of about the same mass, they threw off gravitational waves causing the black holes to lose energy. This loss of energy drew the two black holes closer and closer to each other, ending in a collision. As a result, a new massive black hole, 62 times the mass of the Sun, was created. The lost mass is what created the gravitational energy that sent out waves that were actually detected by the LIGO.
Scientists, for a long time, have been trying to discover these waves—for over 40 years, to be precise. From that point on and with various advancements in technology, they have been able to see more and more of them. Previously, gravitational waves have been hard to detect because they happen so far from us. By the time these waves get to us, they only distort space-time at a very small scale which is hard to observe and quantify. This change in space-time is smaller than the width of a proton! Due to this fact and until the LIGO was built, the available instruments were unable to measure these minute shifts in space-time.
LIGO
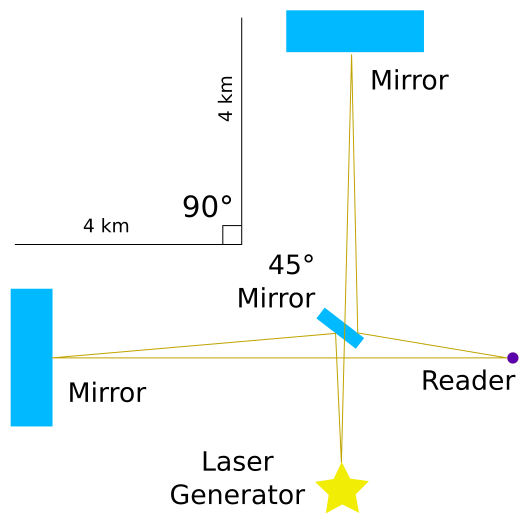
The LIGO has detectors in two “observatories”—in Washington and Louisiana. Each facility as an L-shaped detector that has a laser generator and mirrors that can measure the minutest of changes in space-time. At each observatory, there are two arms 4 kilometers long. A gravitational wave is detected when it slightly changes the length of an arm.
The lasers in the LIGO detectors emit a pulse of light (Laser Generator). The laser hits a beamsplitter (45° Mirror) which sends part of the photons straight and sends others to the left. Now we have to beams going at exactly 90° of each others. The beams bounce on the mirrors at the end of the tunnels and come back to the reader. When there is no gravitational wave, the beams are exactly the same so the light wave match exactly when received by the reader. On the other hand, when a gravitational wave happens, one of the beams is going to be disturbed and the waves will not be aligned at the reader.
This works because we are able to build two tunnels of the exact same length. The detectors are so very sensitive that they detect everything from lightning to passing vehicles, therefore, the same otherwise unexpected discrepancy must be noted by all detectors.
Apart from the LIGO in America, the European Gravitational Observatory’s Virgo detector was recently created and there are plans to have the same in Japan and India. With more observatories to detect these waves, it will soon be possible to identify which specific region a gravitational wave came from.
These devices are extremely important to anyone with a passion for space and the wonders within it. Gravitational waves give us more insight into what happens in space. We are now able to detect large events like the collision of black holes. With knowledge of gravitational waves, scientists have been able to use traditional ways of observing space objects to learn more about celestial bodies such as neutron stars.
Gravitational stars opened up a discussion on the formation of heavy metals on Earth. It was previously speculated that the death of giant stars led to the formation of the gold we have.
With more knowledge on gravitational waves, scientists were able to discover that the joining of neutron stars could end in an explosion called a kilonova that emitted atomic nuclei that resulted in the formation of heavy metals on Earth.
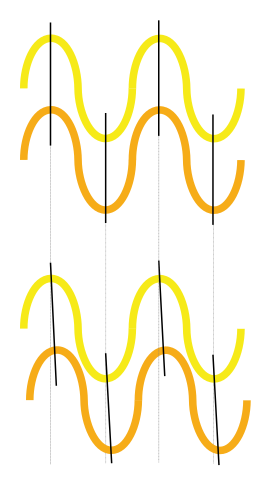
Here is an illustration of what the LIFO reader sees.
When the signal doesn’t get disturbed by anything at all, the light waves from the two signals will match one to one.
If something such as a vehicle generates enough vibration, then we will pick up a difference between both signal: one of them will be early and the other will be late.
When a gravitational wave passes through Earth, one of LIGO’s arms will be affected but not the other. We get the same effect as a vibration, only it will happen at all the LIGO like installations.
As we can see on the picture, the difference between the two signal can be very minimal which is why we need instruments of very high quality to be able to detect anything.
History of the LIGO
American physicists Kip Thorne and Rainer Weiss together with Scottish physicist Ronald Drever had a dream of creating a gravitational wave detector, and they in a sense kicked things off. In 2002, after millions of dollars in funding from the National Science Foundation and years of research, parts of the LIGO were sent to their observatories in Louisiana and Washington. These parts included a 4-kilometer tunnel in an L-shape that had laser beams that were 50,000 times more powerful than a conventional laser. The lasers would bounce from mirrors at opposite ends of the tunnel.
After 8 years, they had still not made any substantial discovery. They ran more tests and calculations on their device, and they discovered that the LIGO did not have the sensitivity needed for it to detect a gravitational wave. It was then shut down in 2010 and US $200 million was given to the team to make necessary upgrades and in 2015, a more sensitive LIGO was released. This new and improved LIGO was able to detect a few quivers within a few days and after a couple of months, it was confirmed that the signal was real. This fete was celebrated by over 1,000 scientists who had worked on the device.
In total, we have been able to discover 23 gravitational-wave events:
- Twenty of these have been black hole mergers,
- Two were neutron star mergers, and
- One of them was a merger between a black hole and a neutron star.
The LIGO was further upgraded in 2018 and 2019 and this improved its sensitivity even further. Some scientists have even stated that by 2023, the LIGO will be able to detect a gravitational wave every hour.
The LIGO and the Virgo were both able to observe the neutron star merger. This merger allowed scientists to study certain aspects of the universe. The telescopes were able to detect gamma rays after the gravitational waves. This allowed scientists to compare the speed of gravity versus the speed of light. The telescopes had detected the gamma rays 1.6 seconds after the gravitational waves. This was proof that the speed of light and the speed of gravity were the same further proving the theory of general relativity.
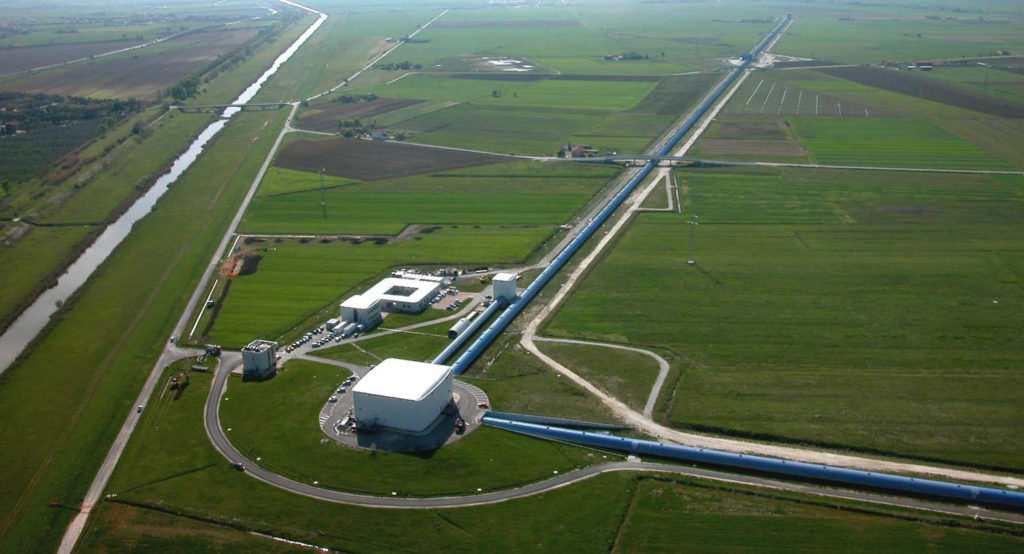
Leave a Reply