All the information on the universe and how it operates comes from our knowledge of light. Light constitutes waves – electromagnetic waves to be specific.
Similar to how a prism splits light into a rainbow (multiple colors) is how spectroscopy splits electromagnetic radiation into different wavelengths which are known as a spectrum. A spectrum is more complicated than a rainbow of colors. While a rainbow is simply different colors that make up white light, electromagnetic radiation constitutes wavelengths that carry a lot of information on the nature of various astronomical objects.
Our eyes can’t tell the difference between minute differences in the shade of a color, however, a spectrometer can spot it. This is because a spectrometer can accurately measure the wavelength of light and can tell the difference between light that is emitted from a hydrogen atom to light that is emitted from helium. As a result, when connected to a telescope, astronomers can figure out what celestial objects are made of.
Spectroscopy allows astronomers to think beyond the visible. It has been said that if we are ever to discover signs of alien life in the universe, then we will discover it through spectroscopy.
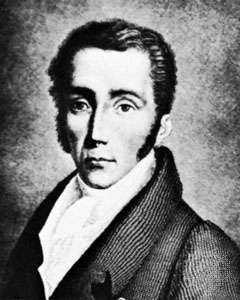
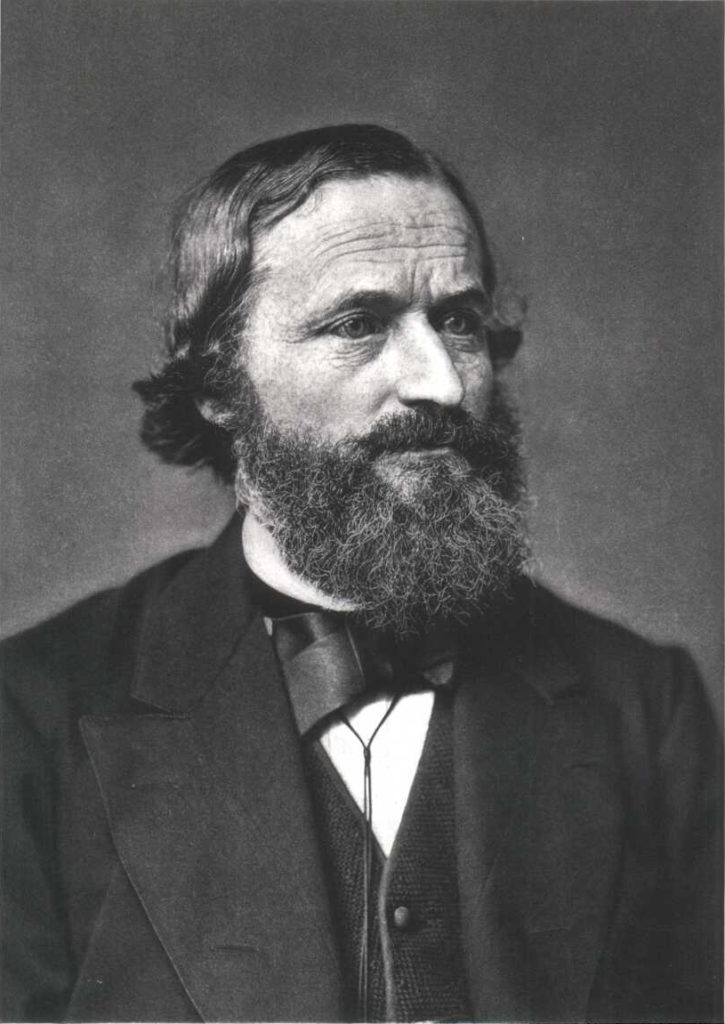
The first use of spectroscopy in astronomy was in the 19th century by Fraunhofer and Kirchhoff. They were analyzing the colors of light when passing through a prism. As expected, they saw a rainbow of light, however, they also noticed that there were dark lines. These dark lines were imprints of chemicals that reacted with the light and are referred to as chemical lines. This discovery showed that each chemical, or more specifically each element of the periodic table, creates its own unique signature in the spectrum. This signature is like a fingerprint of sorts that distinguishes one element from the other. By understanding each fingerprint, astronomers are able to understand the different components that make up any body that absorbs or emits light.
For clouds of gas in space, their atoms are roaming freely, and rarely do they come into contact with one another. In most cases, we see lights from stars behind these clouds. In some cases, the atoms emit their own light because they are ionized. This light is what enables astronomers to identify their composition. For dense stars, their colors depend on their temperature while in the case of thinner gas, what’s in them is what counts. Using this knowledge is how we were able to figure out what the universe is constituted of. Stars and gases are mostly made up of hydrogen and helium and a few other heavier elements.
As mentioned, spectrographs are more complex than prisms. The spectrum of light that comes from a spectrograph is more complex than a visible rainbow. The breaking down of light from these devices is spread more. Once the light is emitted, it is saved on a CCD detector, saved in computer files for later analysis. The spectrum of a star or any other celestial object shows more than the chemical elements of that body. It also shows physical properties such as temperature and density.
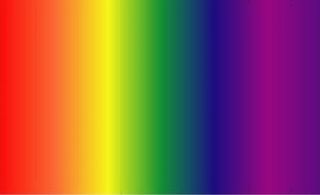
Using the Doppler Effect, spectroscopy can also be used to determine the speed of a star or a galaxy relative to the Earth.
The Doppler Effect
You’re probably familiar with the Doppler Effect in relation to sound. Sound waves are often higher pitched when they are close to us or approaching us. The further away they move from us, the lower-pitched the sound gets. i.e. the sound emitted by the siren of an ambulance approaching and then moving away changes. The reason for this is due to the fact that sound waves are compressed when they approach and stretched out the further away they move. Therefore, a shorter wavelength means a higher pitch and a longer wavelength means a lower pitch.
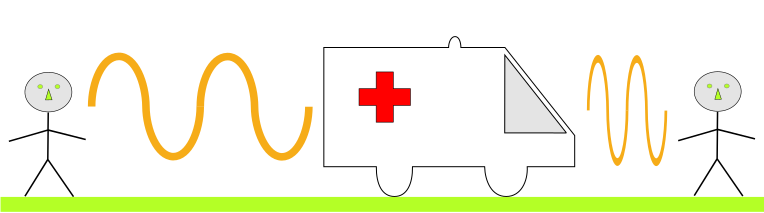
Though light moves much faster than sound, the principle behind this phenomenon remains the same in both instances. When a source of light approaches us, it appears brighter because the wavelength is shorter and it appears dimmer when getting further away from us because the wavelength is long.
Light from a source that is moving towards us is said to be blue-shifted. This is because its wavelength is shorter in the blue region while a light source moving away from us appears to be red-shifted because its wavelength is longer and is in the red region.
Using this knowledge, spectroscopy can also be used to measure the motion of celestial bodies. They can measure the movement of stars closer to us and interstellar bodies such as other galaxies by measuring changes in the wavelength of emission lines. When a celestial body is close to us, its wavelength will be shorter and will appear to be blue-shifted. The further away it is, the longer the wavelength and therefore it will be red-shifted. This is due to the movements in the universe. Objects closer to us tend to come toward us and objects further away tend to go away because of the expansion of the universe.
When Measuring Density
Astronomers often calculate density in the electromagnetic spectrum. This is the number of free electrons within a particular volume. Astronomers calculate electron density by calculating the ratios of the intensities of certain emission lines and comparing this against theoretical values. In simple terms, they measure density by comparing how bright certain emission lines are in comparison to others.
Temperature And Size
We know that an opaque body, or rather a black body can emit electromagnetic radiation at all wavelengths. It is said that by measuring the highest temperature of a star, we’re able to infer its temperature.
This means that objects at different temperatures emit spectra at very diverse wavelengths. Hotter objects have shorter wavelengths and there are more blue-shifted while colder bodies have longer wavelengths and are more red-shifted.
A hotter object typically radiates more than a cooler object.
However, to properly infer one of the temperature, size, or distance, we need two of these parameters. This is why once in a while we find a galaxy which is so far away that it may look older than the universe itself. This is because at such a distance we really only get one of the parameters and the others are really difficult to calculate. For example, GN-z11 has a spectrometric redshift of 11.09. This means it would be at 32 billion years away from here. Since the universe is about 13.4 billion years, nothing can be further away than that… our current technology is just not yet sufficiently precise for those far away galaxies.
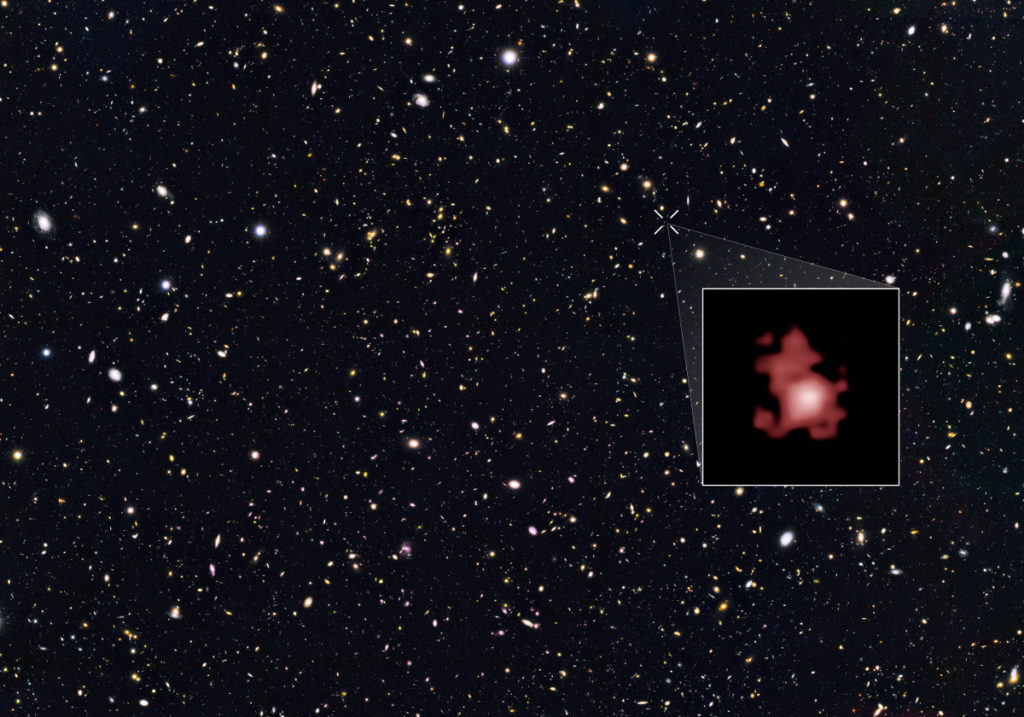
What We’ve Been Able To Observe
Dark Matter
Through spectroscopy, we have been able to study the structure of our universe. We have been able to identify dark matter by studying unexpected speeds of objects at different distances from our galaxy.
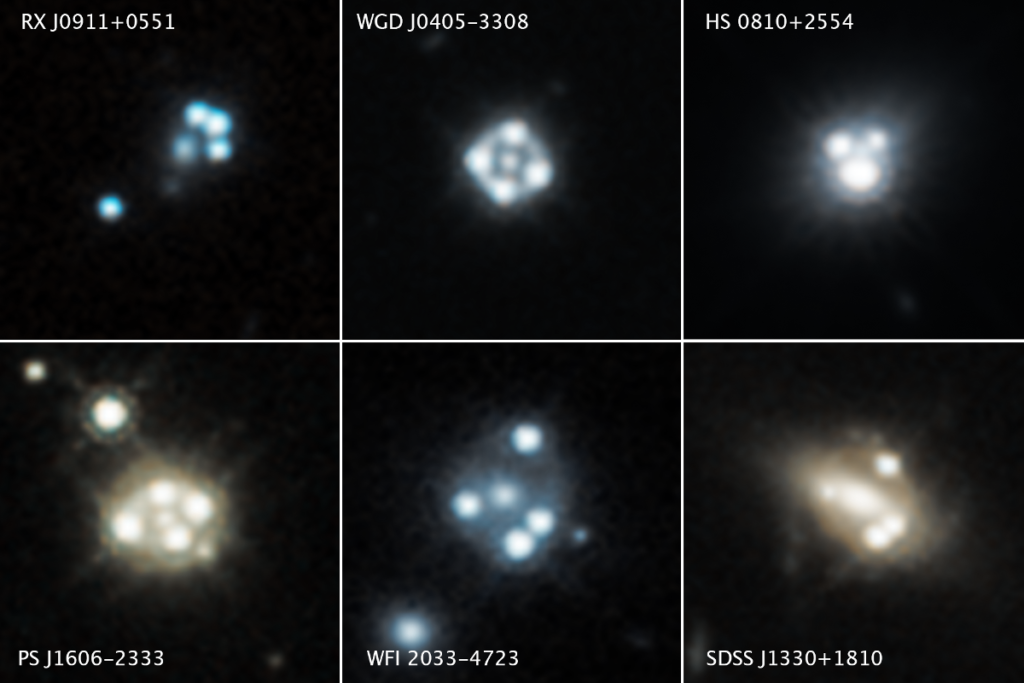
This discovery was first made in the year 1937 by Zwicky, Fritz. While conducting Doppler shift studies, he noticed that galaxies in a cluster seemed to be moving much faster than what was thought to be possible from their mass which was calculated through spectroscopy. He then came to the conclusion that there was matter within the galaxy that did not emit light and quite a substantial amount of it. This has led to astronomers concluding that within galaxies, there is a great deal of dark matter.
Since then we found that dark matter also exists between galaxies.
Four galaxies, however, have been excluded from that theory: NGC 821, NGC 3379, NGC 4494, and NGC 4697. This is problematic since this means dark matter is really not evenly distributed in the universe, making it even more difficult to explain.
Quasars
Quasars are essentially galaxies that are powered by supermassive black holes due to the energy emitted from them. The first quasar was discovered when strong radio waves were seen to come from very dim and red objects. Upon further analysis of the spectra, it was found that absorption lines were seen where they typically should not be found. These radio sources were named quasi-stellar radio sources.
In 1964, Hong-Yee Chiu gave us the name quasar for those objects.
Strange Motions of Galaxies
NGC 4550, a galaxy in the Virgo Cluster, was observed and we noticed that a group of its stars was moving in the opposite direction to the larger majority of stars. It was theorized that two different smaller galaxies were rotating in opposite directions to each other.
Planets
Spectroscopy has been helping us with discovering many exoplanets. Planets contain minerals. When light is reflected off a planet, it contains otherwise unusual absorption bands and that is typical of rocks and rocky bodies. Other elements have also been discovered on these planets and these include water vapor (H2O), carbon dioxide (CO2), carbon monoxide (CO), ozone (O3), sodium (Na), ammonia (NH3) and methane (CH4). In other words, planets that are likely to have an atmosphere similar to ours.
Note that we think that O3 is a strong indicator that there is life on that planet. We’ve already found several such planets!
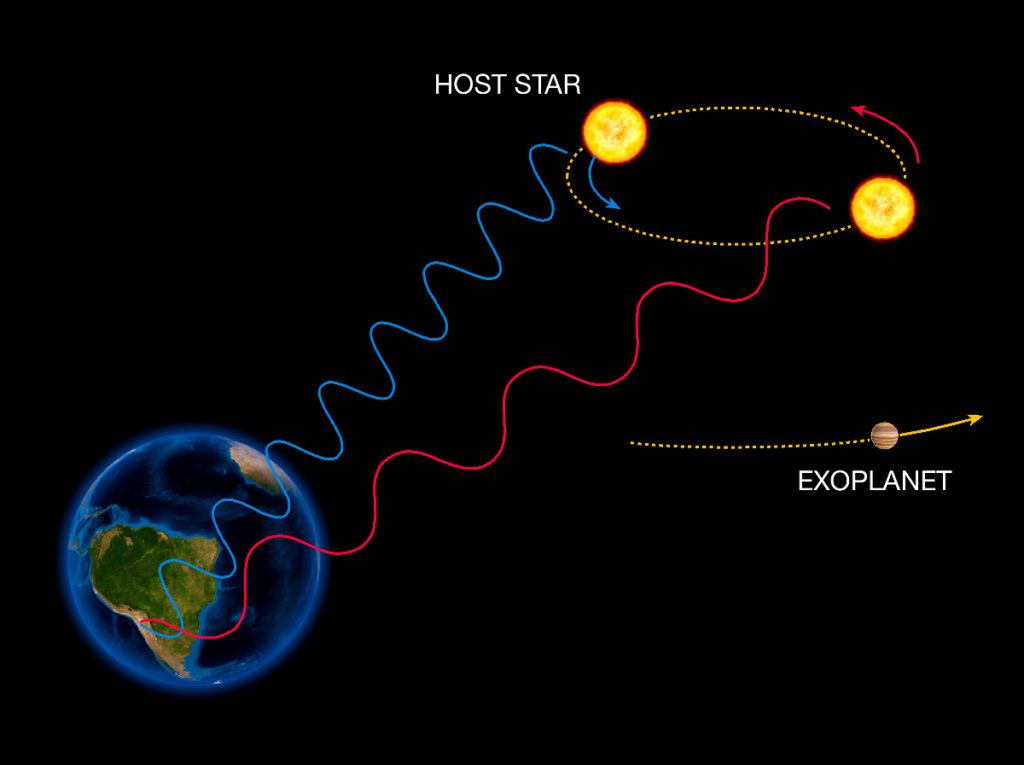
Asteroids
Spectra and spectroscopy have been instrumental in classifying asteroids into three major groups. This process is known as the Tholen classification. The three types are:
- C-types made up of materials primarily composed of carbon
- S-types made up of silicate minerals
- X-types made up of metallic substances
We get a spectra from the solar system asteroids because they reflect light from our Sun and in those reflected beams of light, we can see various bands that are missing. Those bands represent the atoms that those asteroids are made of.
Comets
The spectra of comets consists of a reflected solar spectrum that comes from dusty clouds around these comets. The atoms and molecules within comets also emit emission lines that create a fluorescence when they interact with sunlight and other chemicals.
We assumed that the composition of the core of comets is similar to their tail and the visit of one comet by a European spacescraft, we got a partial confirmation that this is correct.
Expansion Of The Universe
Through spectroscopy, we have been able to understand the expansion of our universe. Edwin Hubble discovered that galaxies that were further away from the Earth have spectra that were red-shifted, while those closer had blue-shifted spectra.
Study Of Binary Stars
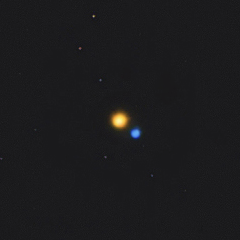
By calculating radial velocity in some orbits through spectroscopy, we can understand more about binary stars. Once we have this knowledge, we can compare it to Newton’s law on gravity or even Kepler’s Law. With additional information on eclipses, we are also able to measure the mass of these stars and even the radii of these stars.
Understanding the Origin of the Milky Way Galaxy Clouds of Gas
Clouds of gas that create a halo around our and other galaxies hold enough matter to birth many new stars. These clouds are high-velocity and are commonly referred to as HVC of gas. Using spectrographs, astronomers from the University of Notre Dame went ahead and measured how big these clouds were.
They first decided to find out how far these clouds were and they did this by observing stars that were surrounded by these clouds of gas. They found that they were 35,000 light years away. They then determined that the mass of these gas clouds was approximately a hundred million times the mass of the Sun and that mass was dropping at one solar mass per year.
Astronomers think that these clouds of gas come from within our Milky Way. When supernovas explode, they do so with so much speed that gas was pushed out of our galaxy and they banded together to form clouds.
We have also found many clouds of gas around other galaxies with similar properties.
All Radiations Generate a Spectroscopy
We first found spectroscopy with visible light.
We then noticed it worked with invisible light as well, such as infrared and ultra violet.
Now that we’ve detected gravitational waves, we can also see spectroscopy in action in those waves.
Astrochemistry
As mentioned spectroscopy is integral to astronomy. Apart from measuring densities, wavelengths, and motion, the chemical composition of materials on Earth can be compared to make inferences on the chemical composition of celestial bodies, the temperature of stars, and interstellar clouds.
Celestial bodies all have molecules formed of atoms and ions that each have specific spectra. This knowledge can help us understand just what elements are in a particular body. However, our types of radiation are only able to detect specific molecular species and those outside these molecular species cannot be identified without going on site.
There has been a lot of research lately into recording the spectra of interstellar mediums. This is the space between stars. Interstellar space is not a complete vacuum and using astrochemistry, scientists have been able to find certain elements including C60 fullerene and water and methanol. There are still attempts to conclusively understand the presence of polycyclic aromatic hydrocarbons (PAHs) in space – which was found in abundance. We are still not able to understand how they form. How would we be able to use this knowledge to understand the physical conditions in the universe? And how they are involved in star formation?
Observatories that are able to conduct this type of spectroscopy include infrared space telescope Spitzer, stratospheric telescope SOFIA or the ground-based observatory ALMA.
Leave a Reply